Everyday Phenomena: Discovering the Physics in Your Daily Life
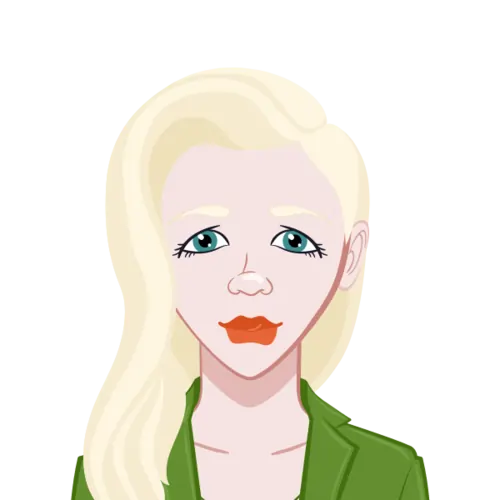
Have you ever wondered about the science behind the seemingly ordinary events that unfold in your daily life? From the moment you wake up to the time you go to bed, there are countless occurrences that are governed by the laws of physics. In this journey of exploration, we will delve into the fascinating world of everyday phenomena and unveil the physics that makes them possible. Whether you require assistance with your physics assignment or simply seek to deepen your understanding of the fundamental principles that underpin our daily experiences, join us as we unravel the mysteries of the universe one phenomenon at a time.
Everyday life is filled with seemingly ordinary occurrences that often go unnoticed, yet beneath the surface, there exists a captivating world governed by the principles of physics. "Everyday Phenomena: Discovering the Physics in Your Daily Life" invites us to embark on a journey of exploration, unveiling the science that shapes our world from the moment we wake up to the time we go to bed. One of the most enchanting aspects of this exploration is the magic of sunrise. As the sun graces the horizon with its presence, the physics of light and the Earth's rotation come into play, orchestrating a symphony of colors that paint the sky each morning. This daily spectacle is a testament to the intricate dance between celestial bodies and atmospheric conditions.
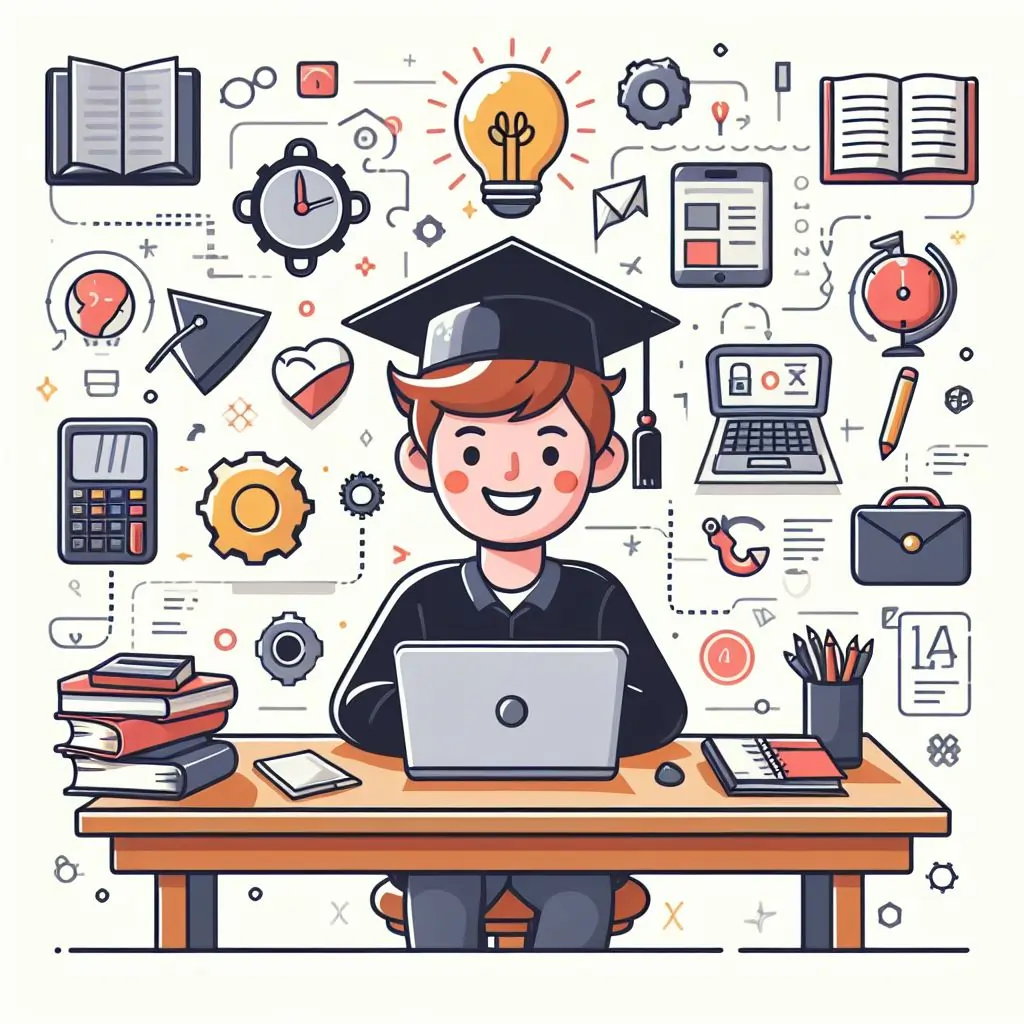
But the physics of our daily existence extends far beyond the celestial ballet of sunrise. Consider the simple act of pouring a cup of coffee, a ritual for many to kickstart their day. This seemingly mundane task unfolds as a complex dance of fluid dynamics. The viscosity of the coffee, the shape of the mug, and the pour itself all contribute to the mesmerizing patterns that form on the surface of the liquid. It's a harmonious blend of physics and routine, where the laws governing the behavior of fluids come to life in the most ordinary of actions.
Walking, a fundamental aspect of human mobility, is another daily phenomenon intimately connected with physics. From the distribution of forces in our joints to the delicate balance required for a smooth stride, biomechanics and Newtonian physics play a crucial role in every step we take. Exploring the mechanics of walking unveils a world of intricate forces and movements that our bodies effortlessly execute, illustrating the elegance of physics in the most basic human activities.
As the seasons change, so too do the phenomena that shape our daily experiences. The dance of autumn leaves, for instance, is a captivating display of nature's transition. The transformation of green foliage into a mosaic of reds, yellows, and browns involves the physics of pigments, sunlight, and changing temperatures. Understanding why leaves change color and gracefully fall from the trees adds a layer of appreciation for the seasonal transitions that surround us.
Rain, often seen as a mundane weather event, transforms into a symphony of physics when examined closely. The journey of raindrops from clouds to the ground involves a series of intricate processes governed by atmospheric conditions. The size and speed of raindrops, the soothing sound of rain tapping on surfaces—all of these elements weave together in a delicate choreography orchestrated by the principles of physics. Even the most common rainy day becomes a canvas for scientific wonders when viewed through the lens of understanding the physics at play.
In essence, "Everyday Phenomena: Discovering the Physics in Your Daily Life" serves as an invitation to embrace the curiosity within and recognize the science inherent in our daily experiences. It is a reminder that the world around us is a stage for the intricate dance of physics, where the seemingly mundane transforms into a canvas for scientific wonders waiting to be uncovered. Whether it's the rising sun, the falling rain, or the simple act of pouring coffee, each moment becomes an opportunity to delve into the underlying principles that govern our existence.
This exploration not only deepens our understanding of the world but also fosters a sense of awe and appreciation for the everyday phenomena that surround us. It encourages us to view our daily experiences through a scientific lens, fostering a curiosity that transforms routine into discovery. "Everyday Phenomena" is not just a title; it's an invitation to rediscover the magic in the ordinary and appreciate the beauty of the physics that shapes our daily lives. So, let us embark on this journey together, where the world we thought we knew reveals itself as a playground for the marvels of physics, waiting to be explored and appreciated in the tapestry of our everyday existence
The Dance of Sunlight: Understanding Sunrise and Sunset
Discover the intricate physics behind the breathtaking moments of sunrise and sunset, from the scattering of sunlight to the Earth's atmosphere. The rising and setting of the sun, a daily occurrence that graces our world with light and warmth, is a mesmerizing phenomenon that captivates observers across the globe. The intricate dance of sunlight during sunrise and sunset is a testament to the profound physics at play in our atmosphere and beyond.
As the Earth rotates on its axis, different parts of the planet are exposed to the sun's rays, creating the cycle of day and night. During sunrise, the first light of the day emerges as the sun ascends above the horizon. This seemingly simple event is a result of the Earth's axial tilt and its orbit around the sun. The changing angles of sunlight contribute to the kaleidoscope of colors that paint the sky, transitioning from soft hues of pink and orange to the brilliant blue of day.
Understanding the physics behind sunrise requires delving into the properties of light. Sunlight is composed of a spectrum of colors, each with a specific wavelength. When the sun is near the horizon during sunrise, its light passes through a thicker layer of the Earth's atmosphere. This atmospheric layer scatters shorter wavelengths, allowing longer wavelengths such as reds and oranges to dominate the sky. This phenomenon, known as Rayleigh scattering, is responsible for the warm and vibrant colors that define the dawn of a new day.
Similarly, as the sun bids farewell during sunset, the dance of sunlight takes on a different, yet equally enchanting, character. The descending sun casts longer shadows and projects its warm glow across the landscape. The colors shift once again, creating a breathtaking spectacle that marks the transition from day to night. During sunset, the atmospheric conditions continue to influence the path of sunlight, sculpting the sky with hues that evoke a sense of serenity and awe.
Coffee Rings and Capillary Action: The Science in Your Morning Brew
Uncover the physics behind the formation of coffee rings and how capillary action plays a role in this common phenomenon. Coffee rings are a fascinating phenomenon that occurs when a droplet of coffee, or any liquid, dries on a surface, leaving behind a distinctive circular pattern. While this may seem like a simple and common occurrence, there is a wealth of scientific principles at play, with capillary action being a key player in the formation of these intricate patterns. Capillary action is the ability of a liquid to flow in narrow spaces without the assistance of, or against, external forces like gravity. In the case of a coffee ring, when a droplet is deposited on a surface, the liquid begins to evaporate. As the outer edges of the droplet evaporate more quickly than the center, capillary action kicks in, causing the liquid to move from the center towards the edges. This migration of liquid is driven by the interaction between the liquid, the solid surface, and the surrounding air, creating a flow that carries suspended particles—such as coffee particles—towards the outer edge. Consequently, the particles accumulate at the perimeter, resulting in the characteristic dark ring that we observe. This phenomenon has implications beyond the realm of spilled coffee; researchers have harnessed the principles of capillary action and coffee ring formation for applications in fields like microfluidics, where precise control over the distribution of particles is essential. Understanding the science behind coffee rings not only adds a layer of appreciation to our morning brew but also opens the door to innovative technologies that leverage these fundamental principles. So, the next time you savor your cup of coffee, take a moment to marvel at the intricate dance of capillary action and the scientific intricacies at play in every sip.
The Physics of Rainbows: A Splash of Color in Rainy Weather
Explore the optics behind the creation of rainbows, from rain droplets to sunlight, and understand the science that brings vibrant colors to the sky. Rainbows are nature's vibrant display of color, a mesmerizing phenomenon that occurs when sunlight interacts with raindrops in the atmosphere. The physics behind rainbows involves the principles of refraction, dispersion, and reflection, all working in harmony to create this awe-inspiring spectacle. When sunlight passes through raindrops suspended in the air, the light is refracted or bent as it enters the droplet. This bending of light is wavelength-dependent, causing the different colors within the sunlight spectrum to separate. This phenomenon, known as dispersion, is what breaks down white sunlight into its constituent colors—red, orange, yellow, green, blue, indigo, and violet. As the dispersed light continues its journey through the droplet, it undergoes internal reflection off the inner surface of the raindrop. This reflection causes the light to exit the droplet, creating the arc of colors that we perceive as a rainbow. The specific angle at which the light exits the droplet determines the position of each color in the rainbow, with red on the outer edge and violet on the inner edge. Interestingly, rainbows are actually full circles, but when observed from the ground, the horizon obstructs the bottom half, leaving us with the familiar semi-circular arc. Double rainbows occur when light undergoes an additional reflection inside the raindrop, producing a fainter and reversed color sequence above the primary rainbow. The physics of rainbows not only adds a touch of wonder to rainy days but also serves as a vivid reminder of the remarkable ways in which light interacts with the world around us, turning a simple shower into a kaleidoscope of colors.
Balancing Act: The Physics of Walking and Staying Upright
Dive into the biomechanics and physics involved in the simple act of walking, maintaining balance, and avoiding stumbling. Walking may seem like a simple and everyday activity, but from a physics perspective, it involves a delicate and intricate balancing act. The human body's ability to maintain an upright position while in motion is a result of complex interactions between biomechanics, neural control, and the laws of physics.
One key aspect of walking is the concept of the center of mass. The center of mass is the point in a body where its mass can be considered to be concentrated. For a standing person, the center of mass is typically located around the hips. When walking, the body continually shifts its weight from one foot to the other, causing the center of mass to move in a rhythmic pattern. To stay upright, the body must ensure that the center of mass remains within the base of support, defined by the area between the two feet. This alignment is crucial for stability.
Moreover, the swinging motion of the legs during walking introduces another challenge: maintaining angular momentum. As each leg swings forward, the body experiences a rotational force. To counteract this, the upper body subtly twists in the opposite direction, allowing the body to maintain balance and prevent falling. This action showcases the conservation of angular momentum in action.
The nervous system also plays a vital role in the physics of walking. The brain receives constant feedback from sensory receptors, such as those in the inner ear, providing information about the body's orientation in space. The brain processes this information and sends signals to muscles, adjusting their activity to maintain balance. This intricate feedback loop occurs in real-time, allowing individuals to make rapid and automatic adjustments to prevent falling.
Understanding the physics of walking goes beyond the mechanics of leg movement; it delves into the intricacies of biomechanics, stability, and neural control. Whether strolling casually or navigating challenging terrains, the human body seamlessly integrates these principles to execute a remarkable balancing act, showcasing the remarkable synergy between physics and the biomechanics of everyday activities.
The Hum of Electricity: Decoding the Physics of Power
Delve into the world of electricity and discover the physics behind the hum of power lines, sparking a new appreciation for the energy that powers our daily lives. The hum of electricity is a ubiquitous symphony in our modern lives, emanating from the myriad devices and power sources that surround us. Delving into the physics of power reveals a complex interplay of principles governing the generation, transmission, and consumption of electrical energy.
At its core, electricity is the flow of electrons, and the physics governing this phenomenon can be explained by Ohm's Law. This fundamental principle, formulated by Georg Simon Ohm, states that the current (I) flowing through a conductor between two points is directly proportional to the voltage (V) across the two points, with the proportionality constant being the electrical resistance (R). This relationship is expressed as I = V/R. Understanding Ohm's Law is essential for engineers and physicists in designing and optimizing electrical circuits.
The generation of electricity often involves the conversion of mechanical energy into electrical energy, as seen in power plants. In hydroelectric plants, for instance, the movement of water turns turbines connected to generators, producing electrical energy. In thermal power plants, heat is used to generate steam, which in turn drives turbines. These processes adhere to the principles of thermodynamics and electromagnetic induction, showcasing the conversion of energy between different forms.
As electricity is generated, it must traverse an intricate network of transmission lines and transformers. The physics of electromagnetism plays a crucial role in this journey. Alternating current (AC), the standard for electrical transmission, is employed to minimize energy loss during long-distance travel. Transformers change the voltage levels to facilitate efficient transmission, as lower currents at higher voltages result in reduced energy loss due to electrical resistance.
The hum of electricity is also intimately linked to the behavior of semiconductors in electronic devices. The physics of semiconductors, governed by quantum mechanics, enables the creation of transistors, diodes, and integrated circuits. These components form the backbone of modern electronics, allowing for the creation of computers, smartphones, and a vast array of technological marvels.
In essence, the hum of electricity is a manifestation of the intricate dance of electrons, guided by the principles of electromagnetism, quantum mechanics, and thermodynamics.
Floating on Water: The Science of Buoyancy
Learn how the principles of buoyancy govern the ability of objects to float or sink in water, from ships on the ocean to ice cubes in your drink. The seemingly magical ability of objects to float on water is grounded in the fundamental principle of buoyancy, a concept deeply rooted in the physics of fluid mechanics. At the heart of this phenomenon is Archimedes' principle, named after the ancient Greek mathematician and inventor Archimedes, who discovered it over two thousand years ago.
Archimedes' principle states that an object submerged in a fluid experiences an upward buoyant force equal to the weight of the fluid it displaces. This buoyant force counteracts the gravitational force acting on the object, allowing it to float or, in some cases, become partially submerged. This principle provides the explanation for why a steel ship, which is much denser than water, can stay afloat—the ship's shape allows it to displace a volume of water with a weight equal to its own.
The key to buoyancy lies in the concept of density. An object will float if its average density is less than the density of the fluid it displaces. Conversely, if the object is denser than the fluid, it will sink. This principle is evident in the design of boats and ships, where materials are strategically distributed to ensure the vessel's overall density is lower than that of water.
The buoyant force is also responsible for the sensation of weightlessness experienced by objects and even humans when submerged in water. The upward force counteracts gravity, making the effective weight of the object or person less than it would be in the absence of buoyancy.
The science of buoyancy has practical implications beyond understanding why certain objects float. It plays a crucial role in various engineering applications, such as the design of submarines, hot air balloons, and life vests. Engineers and architects use principles of buoyancy to create structures that can float or submerge strategically, depending on their intended function.
In summary, the science of buoyancy, anchored in Archimedes' principle, provides a profound understanding of why objects float or sink in fluids. It showcases the delicate balance between gravitational forces and buoyant forces, allowing us to marvel at the physics behind the everyday phenomenon of floating on water
Mirrors and Reflections: The Optics Behind What You See
Explore the physics of mirrors and reflections, understanding how light interacts with surfaces to give us clear and distorted images. Mirrors, those ubiquitous objects in our daily lives, hold more than just a reflective surface; they are portals into the world of optics, where the behavior of light shapes our perception of the world. The science behind mirrors and reflections is grounded in the principles of optics, a branch of physics that deals with the properties and behavior of light.
The surface of a mirror is typically coated with a thin layer of metal, usually aluminum or silver, that reflects light with minimal absorption. When light waves encounter this smooth surface, they undergo a process called specular reflection. Specular reflection involves the reflection of light waves in a specific direction, preserving the angle of incidence and the angle of reflection. This precise reflection is what allows mirrors to faithfully reproduce the images of objects in front of them.
Understanding mirrors involves grasping the concept of the focal point. In a concave mirror, which curves inward, parallel rays of light converge at a focal point. Conversely, in a convex mirror, which curves outward, parallel rays appear to diverge from a virtual focal point behind the mirror. These properties contribute to the varied applications of mirrors in optical systems, from makeup mirrors to astronomical telescopes.
The law of reflection, a fundamental principle in optics, states that the angle of incidence is equal to the angle of reflection. This law governs the behavior of light as it bounces off mirrors and is crucial in explaining the formation of images in flat and curved mirrors. Flat mirrors produce virtual images that appear behind the mirror, while concave and convex mirrors create real or virtual images based on the location of the object relative to the mirror's focal point.
Mirrors also play a pivotal role in constructing optical devices like periscopes, cameras, and lasers. The precise manipulation of light through reflection allows engineers to design instruments that capture and manipulate images, showcasing the seamless integration of optics into our technology-driven world.
In essence, mirrors and reflections serve as a captivating intersection of physics and everyday experience. From the simple act of checking one's reflection to the intricate workings of advanced optical instruments, the science of mirrors opens a window into the captivating world of optics, where the behavior of light shapes the way we perceive and interact with our surroundings
Swinging Pendulums: The Physics of Timekeeping
Unravel the connection between pendulum motion and timekeeping, tracing the roots of this simple physics phenomenon to the heart of clock mechanisms. Swinging pendulums are not just elegant displays of motion; they are fundamental components of timekeeping mechanisms that have played a crucial role in our understanding and measurement of time. The physics of swinging pendulums is deeply rooted in classical mechanics and provides the basis for various timekeeping devices, including grandfather clocks and pendulum clocks.
The motion of a swinging pendulum is governed by simple harmonic motion, a type of periodic motion characterized by a restoring force proportional to the displacement from equilibrium. In the case of a pendulum, the restoring force is gravity, which pulls the mass (bob) back towards its equilibrium position. The period of a pendulum, the time it takes to complete one full swing, is determined by factors such as the length of the pendulum and the local acceleration due to gravity.
The formula for the period of a simple pendulum, often attributed to Galileo Galilei, is T = 2π√(L/g), where T is the period, L is the length of the pendulum, and g is the acceleration due to gravity. This formula highlights the inverse relationship between the period and the square root of the length of the pendulum, demonstrating that longer pendulums have longer periods.
The remarkable feature of pendulum clocks lies in their ability to maintain accurate timekeeping over extended periods. This is achieved through the isochronous property of the pendulum, meaning that the period of oscillation is nearly constant, regardless of the amplitude of the swing. This property is a consequence of the restoring force being directly proportional to the displacement, a characteristic of simple harmonic motion.
Pendulum clocks typically incorporate escapements, which are mechanisms that control the release of energy from the clock's power source to the pendulum. The escapement alternately allows and restrains the motion of the pendulum, ensuring that it swings with a regular and precise rhythm. This rhythmic motion becomes the basis for timekeeping, with each swing of the pendulum marking a unit of time.
While modern timekeeping has transitioned to more advanced methods, such as quartz crystals and atomic clocks, the physics of swinging pendulums remains a cornerstone in the history of timekeeping. The elegance and simplicity of pendulum motion have not only contributed to the development of accurate timekeeping devices but have also left an indelible mark on the way we perceive and measure time in our daily lives.
Sound Waves in Your Ear: The Physics of Hearing
Discover how sound waves travel through the air and interact with your ears, leading to the amazing ability to hear the world around you. The experience of sound is a remarkable interplay of physics within the intricate anatomy of the human ear. Sound waves, which are oscillations of air pressure, initiate this journey by traveling through the ear canal. The outer ear serves as a funnel, channeling these waves towards the eardrum. The eardrum, a thin membrane stretched tightly across the ear canal, vibrates in response to the incoming sound waves. This vibration sets the middle ear bones - the malleus, incus, and stapes - into motion. The mechanical energy is then transformed into fluid motion within the cochlea, the spiral-shaped, fluid-filled organ of the inner ear. Here, specialized hair cells detect the vibrations and convert them into electrical signals that travel along the auditory nerve to the brain. The brain interprets these signals as sound, allowing us to perceive the rich tapestry of auditory experiences. The physics of sound waves, from their propagation in the air to their conversion into neural signals, underscores the marvel of hearing and showcases the intricate harmony between physics and biology in our perception of the world.
The Physics of Cooking: Heat, Energy, and Culinary Magic
Explore the science of heat transfer, conduction, and convection in the kitchen, unraveling the physics behind the magic of cooking.
Embark on a journey of discovery as we delve into the everyday phenomena that surround us, unveiling the physics principles at play in the seemingly ordinary moments of our lives. Join us in unraveling the mysteries behind these common experiences and gaining a newfound appreciation for the scientific wonders hidden in plain sight. Cooking, that daily alchemical art, is a symphony of heat, energy transfer, and transformative processes orchestrated by the principles of physics. At its core, cooking involves the manipulation of heat to induce chemical and physical changes in food. The first law of thermodynamics, which states that energy cannot be created or destroyed, but only transferred or converted, is fundamental in understanding how heat is harnessed in the kitchen. When we apply heat to food, energy is transferred, causing molecules to vibrate, and chemical reactions to occur. The Maillard reaction, for example, responsible for the browning of meat and the creation of complex flavors, is a result of heat-induced chemical transformations between amino acids and reducing sugars.
The second law of thermodynamics, which deals with the direction of energy transfer, explains why heat flows from a higher temperature to a lower one. This principle guides techniques such as sautéing, roasting, and boiling, where temperature differentials drive the transfer of heat, changing the texture and flavor of ingredients. Furthermore, the phase transitions of water, from solid to liquid to gas, are critical in cooking. Melting butter, simmering sauces, and steaming vegetables all rely on the absorption or release of heat during these phase transitions.
The physics of conduction, convection, and radiation also play pivotal roles in the culinary realm. Heat conducts through metals and other materials, leading to the sizzle of a steak on a hot grill. Convection currents in liquids and gases distribute heat, as seen in boiling liquids or the circulation of air in an oven. Radiation, the transmission of energy through electromagnetic waves, is harnessed in microwave ovens to cook food by exciting water molecules.
Understanding the physics of cooking not only enables culinary mastery but also unveils the magic behind the art of creating delicious dishes. From the careful control of temperatures to the artful manipulation of energy transfer, every cook becomes a physicist in the kitchen, orchestrating a dance of molecules and energy to transform raw ingredients into delectable meals. The culinary journey, with its flavorful destinations, is a testament to the symbiotic relationship between the science of physics and the enchanting world of gastronomy.
Conclusion
Delving into the physics behind the phenomena that surround us provides a profound insight into the intricacies of the natural world. From the gentle swing of a pendulum keeping time to the vibrant colors of a rainbow painted across the sky, each occurrence is a manifestation of underlying physical principles. The science of buoyancy explains why objects float or sink in fluids, mirroring the delicate balance between gravitational forces and buoyant forces. Meanwhile, the rhythmic motion of a pendulum, governed by the laws of simple harmonic motion, has been harnessed for centuries in timekeeping devices, highlighting the marriage of physics and precision engineering.
As we unravel the science behind mirrors and reflections, we discover the precise laws of reflection and the creation of images, showcasing the elegance with which light behaves. The hum of electricity, omnipresent in our modern lives, is a testament to the principles of electromagnetism and the remarkable synergy between physics and technology. The enchanting play of sound waves in our ears takes us on a journey through the auditory realm, where vibrations and frequencies create the rich tapestry of sounds that form the soundtrack to our lives.
The physics of cooking, a symphony of heat, energy transfer, and molecular transformations, transforms raw ingredients into culinary delights. From the conduction of heat in searing a steak to the convection currents in boiling liquids and the radiation in a microwave oven, every culinary creation is a manifestation of fundamental physical principles. The conclusion drawn from exploring these diverse phenomena is that our world is an intricately connected web of physics, influencing not only the way we perceive our surroundings but also the way we interact with and manipulate the elements of our environment.
In this exploration, we find that physics is not confined to laboratories and equations but is woven into the fabric of our daily lives. Whether we are savoring a cup of coffee, marveling at a rainbow, or cooking a meal, we are constantly engaging with the underlying principles that govern the behavior of the universe. This realization adds a layer of depth and appreciation to our experiences, turning the mundane into the extraordinary and highlighting the beauty that emerges when science and the everyday seamlessly intertwine.