Demystifying Essential Electromagnetic Theory for Students | Unveiling Must-Know Concepts
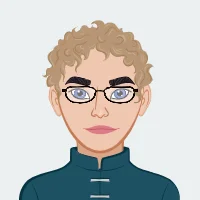
In the intricate realm of physics, understanding the core principles of electromagnetic theory is a paramount journey for students venturing into the fascinating world of science. This comprehensive exploration aims to demystify the essential facets of electromagnetic theory, unraveling the intricacies that often challenge and captivate the curious minds of students. At its foundation lies a historical perspective, tracing the evolution of electromagnetic theory through pivotal discoveries and breakthroughs that have shaped our understanding of the universe. From the fundamental concepts of electric and magnetic fields to the profound significance of Maxwell's equations, this discourse navigates through the theoretical bedrock that forms the backbone of electromagnetic phenomena. If you need assistance with your electromagnetic theory assignment, feel free to seek guidance or clarification on specific concepts presented in this exploration.
As we delve deeper, the discourse transitions into the exploration of electromagnetic waves, elucidating their nature, characteristics, and the profound implications they hold in the broader scientific landscape. Practical applications of electromagnetic theory emerge as a focal point, bridging the theoretical with the tangible, as students are guided through real-world scenarios where these principles find relevance and utility. The narrative further unfolds with a meticulous examination of key experiments that have played pivotal roles in advancing our comprehension of electromagnetic phenomena, offering insights into the empirical foundations that underpin theoretical frameworks.
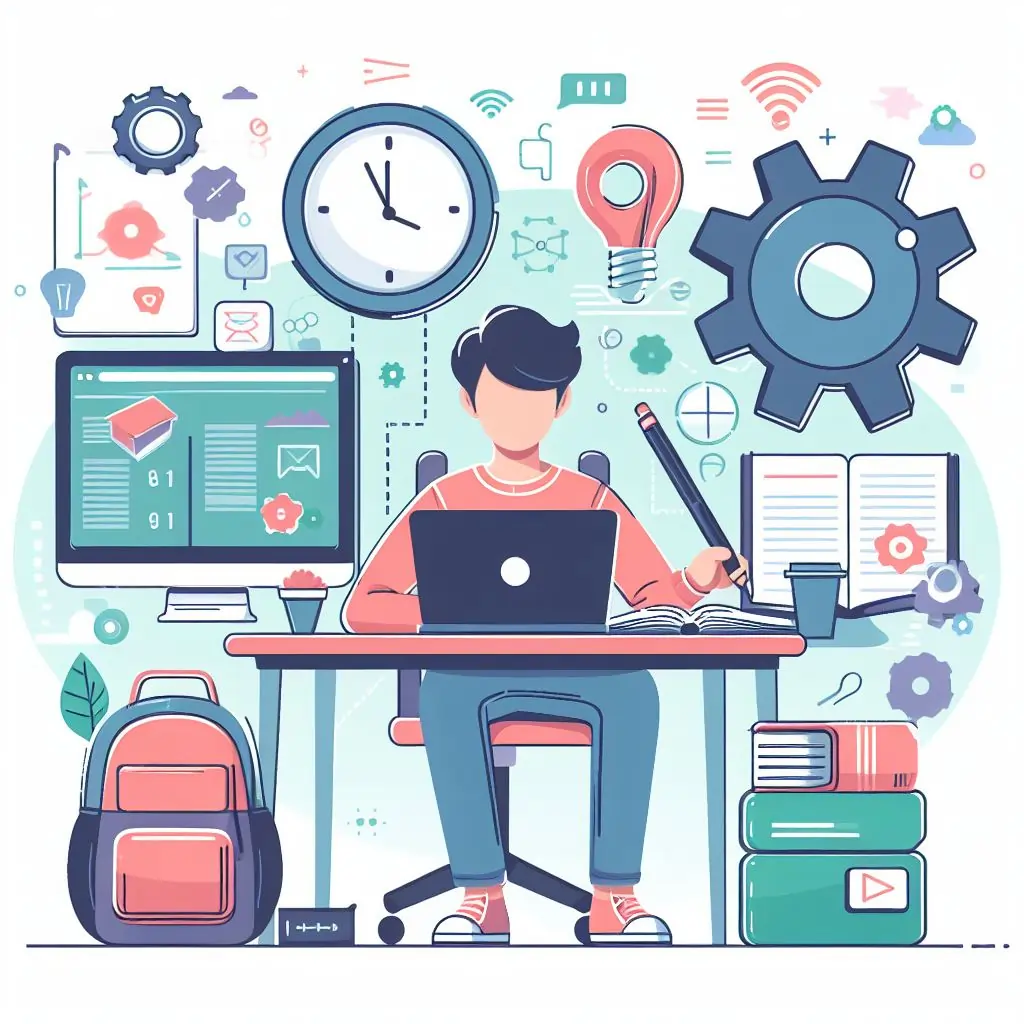
This exploration does not shy away from the mathematical intricacies inherent in electromagnetic theory. It dedicates attention to unraveling the mathematical nuances behind electromagnetic formulas, aiming to simplify complex concepts for students grappling with the equations that define this discipline. Beyond historical perspectives and theoretical frameworks, modern developments in electromagnetic research are highlighted, showcasing the dynamic nature of this field and the exciting frontiers that await exploration.
As students embark on the journey of unraveling essential electromagnetic theory, this discourse not only serves as a guide but also provides valuable tips and resources to aid in the mastery of this intricate subject. With a blend of historical context, theoretical depth, practical applications, and contemporary insights, this exploration stands as a beacon, illuminating the path for students seeking to grasp the must-know concepts of electromagnetic theory.
Historical Perspectives on Electromagnetic Principles
Historical Perspectives on Electromagnetic Principles offer a captivating journey through the annals of scientific discovery, unveiling the rich tapestry of events that led to our current understanding of electromagnetic phenomena. At its genesis, this historical narrative delves into the pioneering work of luminaries such as Michael Faraday and André-Marie Ampère, whose groundbreaking experiments in the 19th century laid the foundation for the development of electromagnetic theory.
The narrative unfolds with Faraday's revolutionary experiments on the relationship between electricity and magnetism, introducing the concept of electromagnetic induction. This watershed moment marked the inception of a new era, demonstrating the interconnectedness of electric and magnetic fields. Simultaneously, Ampère's work on the mathematical formulation of electromagnetism contributed significantly, paving the way for the unification of these two fundamental forces.
Moving forward, the exploration encompasses James Clerk Maxwell's monumental contributions in the mid-19th century, where he synthesized and formalized the disparate findings into a set of equations that would come to be known as Maxwell's equations. These equations, often regarded as the crowning achievement of classical electromagnetism, provided a unified framework, predicting the existence of electromagnetic waves and fundamentally altering our comprehension of the nature of light.
The historical journey doesn't merely recount discoveries but also acknowledges the pivotal role of individuals like Heinrich Hertz, who experimentally verified Maxwell's predictions by generating and detecting electromagnetic waves. This experimental validation solidified the foundation of electromagnetism and paved the way for the technological revolutions of the 20th century.
As the narrative progresses through the 20th century, it explores the quantum mechanical revolution and the synthesis of electromagnetism with quantum electrodynamics, showcasing the continuous evolution of our understanding. Through the lens of historical perspectives, students gain insight into the perseverance, ingenuity, and collaborative efforts that have shaped the electromagnetic principles we now consider fundamental to our understanding of the physical world. Ultimately, Historical Perspectives on Electromagnetic Principles serve not only as a chronicle of scientific progress but as an inspiring testament to the human quest for knowledge and the transformative power of discovery.
Fundamental Concepts: Electric Fields and Magnetic Fields
Fundamental Concepts: Electric Fields and Magnetic Fields delve into the foundational pillars that underpin the fascinating realm of electromagnetism, offering a profound exploration of the essential principles governing the behavior of charged particles and magnetic phenomena.
At the core of this examination are electric fields, conceptualized as regions in space where a charged particle experiences a force due to the presence of other charges. The elucidation of electric fields involves a meticulous examination of Coulomb's law, which quantifies the force between charged particles, and the concept of electric potential, a scalar field that characterizes the energy associated with a given configuration of charges. Understanding these electric field concepts is fundamental for grasping the interactions that govern the behavior of charged particles in diverse physical scenarios.
Simultaneously, the discourse extends its reach to magnetic fields, exploring the intricate dynamics of magnetic interactions. The narrative unveils the contributions of André-Marie Ampère, whose experiments with current-carrying conductors laid the groundwork for comprehending magnetic fields. The concept of magnetic induction, as expounded by Michael Faraday, further enriches the discussion, emphasizing the dynamic relationship between changing magnetic fields and induced electric currents. The interplay of electric and magnetic fields, encapsulated in Maxwell's equations, forms a cornerstone in the elucidation of electromagnetic phenomena.
The exploration of fundamental concepts encompasses the visualization of these fields through field lines, providing a tangible representation of the spatial distribution and strength of electric and magnetic fields. Students are guided through the intricacies of Gauss's law for electric fields and Gauss's law for magnetism, reinforcing the mathematical foundations that govern these phenomena.
As the discourse unfolds, the synergy between electric and magnetic fields comes to the forefront, culminating in the concept of electromagnetic waves. This linkage, central to the unification of electricity and magnetism, underscores the elegance and unity embedded in the fundamental concepts of electric and magnetic fields.
In essence, Fundamental Concepts: Electric Fields and Magnetic Fields offer students a comprehensive journey into the intricacies of these foundational principles, empowering them to comprehend the intricacies of electromagnetic interactions and paving the way for a deeper exploration of the captivating world of electromagnetism.
Maxwell's Equations: The Cornerstone of Electromagnetic Theory
At the pinnacle of electromagnetic theory stands Maxwell's Equations, a set of four interrelated mathematical expressions that encapsulate the fundamental principles governing the behavior of electric and magnetic fields. This conceptual cornerstone, developed by the visionary Scottish physicist James Clerk Maxwell in the mid-19th century, represents a monumental synthesis of prior discoveries and a paradigm shift in our understanding of electromagnetism.
The first of Maxwell's equations, Gauss's Law for Electricity, reveals the relationship between the electric field and the distribution of electric charges. It articulates how the electric flux through a closed surface is proportional to the enclosed charge, laying the groundwork for comprehending the behavior of electric fields in different configurations.
The second equation, Gauss's Law for Magnetism, introduces a complementary perspective by postulating that magnetic monopoles do not exist. It elucidates how magnetic field lines neither begin nor end, emphasizing the closed nature of magnetic flux lines, a concept that distinguishes magnetic fields from electric fields.
Faraday's Law of Induction constitutes the third equation, illustrating how a changing magnetic field induces an electromotive force (EMF) and subsequently an electric current in a closed loop. This dynamic interplay between magnetic fields and induced electric currents forms the basis for various technological applications, from generators to transformers.
Ampère's Law with Maxwell's Addition, the fourth equation, unifies the theories of electricity and magnetism by incorporating the concept of displacement current. This innovative addition, proposed by Maxwell himself, accounts for the changing electric field in regions where there is a time-varying magnetic field, completing the set of equations and paving the way for the prediction and understanding of electromagnetic waves.
Collectively, Maxwell's Equations culminate in the realization that electric and magnetic fields are not independent entities but are intimately connected, oscillating and propagating together as electromagnetic waves at the speed of light. This groundbreaking insight forms the theoretical basis for modern telecommunications, radar systems, and countless other applications that have transformed the technological landscape.
In essence, Maxwell's Equations stand as the bedrock of electromagnetic theory, providing a unified and elegant framework that has withstood the test of time. Their profound implications continue to shape the landscape of physics and engineering, underscoring the power of mathematical abstraction in unraveling the mysteries of the natural world.
Electromagnetic Waves: Nature and Characteristics
Embarking on the captivating journey through the intricacies of electromagnetic theory, the exploration of electromagnetic waves emerges as a pivotal chapter, unveiling the profound nature and distinctive characteristics of these ubiquitous phenomena.
At its essence, electromagnetic waves are rhythmic oscillations of electric and magnetic fields that propagate through space, carrying energy and information. As elucidated by James Clerk Maxwell's groundbreaking equations, the synergy between changing electric fields and magnetic fields results in the self-propagating nature of these waves. A fundamental characteristic of electromagnetic waves is their ability to traverse the vacuum of space, showcasing the universality and omnipresence of these phenomena in the cosmos.
The nature of electromagnetic waves is intricately tied to their frequency and wavelength. The electromagnetic spectrum spans an expansive range, encompassing radio waves, microwaves, infrared radiation, visible light, ultraviolet radiation, X-rays, and gamma rays. Each segment of this spectrum corresponds to waves with specific frequencies and wavelengths, dictating their diverse applications and interactions with matter.
One of the defining features of electromagnetic waves is their speed of propagation, and in a vacuum, they travel at the speed of light, approximately 3 x 10^8 meters per second. This universal constant establishes a cosmic speed limit and underscores the intimate connection between the electromagnetic spectrum and the fundamental constants of nature.
The exploration of the characteristics of electromagnetic waves extends to their polarization, a property that describes the orientation of the electric field vector as the wave propagates. Understanding polarization is essential for various applications, from telecommunications to the functioning of optical devices.
Moreover, the discourse delves into the concept of wave interference and diffraction, illustrating how electromagnetic waves can superimpose or bend around obstacles, leading to intricate patterns and phenomena observed in different contexts.
As the narrative unfolds, the practical implications of electromagnetic waves become apparent, ranging from the transmission of information through radio waves to medical imaging with X-rays. The versatility and pervasiveness of these waves in everyday life underscore their significance in modern technology and scientific exploration.
In summary, the exploration of Electromagnetic Waves: Nature and Characteristics provides students with a comprehensive understanding of the fundamental properties and behaviors that define these waves. From their intrinsic connection to Maxwell's equations to their diverse applications across the electromagnetic spectrum, this exploration forms a cornerstone for comprehending the far-reaching impact of electromagnetic waves on our understanding of the natural world and technological advancements.
Practical Applications of Electromagnetic Theory
The theoretical underpinnings of electromagnetic theory, as laid out by luminaries like James Clerk Maxwell, come to vibrant life through a myriad of practical applications that have revolutionized the modern world. This exploration delves into the tangible and transformative ways in which electromagnetic principles are harnessed across various fields.
- Communication Technology: At the forefront of practical applications lies the realm of communication technology. Radio waves, microwaves, and infrared waves, all integral components of the electromagnetic spectrum, form the backbone of wireless communication. From radio and television broadcasting to satellite communication and cellular networks, electromagnetic waves enable the seamless transmission of information over vast distances.
- Medical Imaging: Electromagnetic theory plays a pivotal role in the field of medical diagnostics. X-rays, a form of high-energy electromagnetic radiation, are employed for imaging internal structures in medicine. Magnetic Resonance Imaging (MRI) relies on the principles of electromagnetic resonance to create detailed images of soft tissues, providing invaluable insights for medical practitioners.
- Power Generation and Transmission: The generation and transmission of electrical power, fundamental to modern society, hinge on the principles of electromagnetic induction. Generators convert mechanical energy into electrical energy through the motion of magnets within magnetic fields. Transformers, utilizing electromagnetic induction, facilitate the efficient transmission of electrical power over long distances.
- Electromagnetic Compatibility (EMC): In the realm of electronics, electromagnetic compatibility is crucial to ensure that different devices can coexist without interference. Understanding electromagnetic theory is essential for designing electronic systems and devices that operate harmoniously in a shared environment, preventing unwanted electromagnetic interference.
- Wireless Technologies: Beyond traditional communication, electromagnetic theory forms the basis for an array of wireless technologies. Bluetooth and Wi-Fi, for instance, leverage radio waves to enable seamless connectivity between devices. Near Field Communication (NFC) utilizes electromagnetic fields for short-range communication, finding applications in contactless payments and data transfer.
- Radar Systems: Radar, an acronym for Radio Detection and Ranging, relies on the principles of electromagnetic waves to detect and locate objects. By emitting radio waves and analyzing their reflections, radar systems are instrumental in applications ranging from air traffic control and weather monitoring to military defense.
- Electromagnetic Sensing: Electromagnetic sensors are employed in diverse fields for a range of applications. Magnetic sensors, for instance, are integral to compasses and navigation systems. Electromagnetic sensors also find use in detecting metal objects in security screening and industrial settings.
- Renewable Energy: Electromagnetic principles are harnessed in renewable energy technologies. Solar panels utilize the photoelectric effect, an electromagnetic phenomenon, to convert sunlight into electricity. Wind turbines, through the rotation of magnets within magnetic fields, generate electrical power.
- Electromagnetic Levitation: Magnetic levitation technology, utilized in high-speed maglev trains and certain industrial processes, relies on the repulsion and attraction forces generated by electromagnetic fields to lift and propel objects without physical contact.
- Electromagnetic Research Tools: In scientific research, electromagnetic theory underpins a plethora of experimental tools. Particle accelerators, such as those in high-energy physics, employ magnetic fields to control the trajectory of charged particles. Electromagnetic spectrometers and detectors play crucial roles in studying the properties of matter and the cosmos.
In essence, the practical applications of electromagnetic theory permeate nearly every facet of contemporary life, showcasing the transformative impact of understanding and harnessing the fundamental principles elucidated by Maxwell's equations. From the seamless flow of information to advancements in medical diagnostics and the generation of clean energy, the practical manifestations of electromagnetic theory underscore its indispensable role in shaping the technological landscape.
Key Experiments that Shaped Electromagnetic Understanding
The evolution of electromagnetic theory is intricately woven with a series of groundbreaking experiments that have illuminated the fundamental principles governing the interaction between electric and magnetic fields. These key experiments, conducted by pioneering scientists over the centuries, have played a pivotal role in shaping our understanding of electromagnetism and have paved the way for the technological advancements that define the modern era.
- Coulomb's Experiment (1785): Charles-Augustin de Coulomb's meticulous experiments with charged objects laid the groundwork for understanding the fundamental principles of electric fields. His observations and quantification of the force between charged particles, as detailed in Coulomb's Law, provided a crucial foundation for later developments in electromagnetic theory.
- Ampère's Experiment (1820): André-Marie Ampère's experiments with current-carrying conductors demonstrated the magnetic effects of electric currents. Through his investigations, Ampère established the relationship between current and magnetic fields, formulating Ampère's Law, a key contribution to the unification of electricity and magnetism.
- Faraday's Induction Experiments (1831): Michael Faraday's groundbreaking experiments on electromagnetic induction laid the groundwork for understanding the relationship between changing magnetic fields and induced electric currents. Faraday's Law of Induction, formulated as a result of these experiments, became a cornerstone of electromagnetic theory.
- Maxwell's Equations (1861-1865): James Clerk Maxwell's synthesis of prior experimental and theoretical work resulted in the formulation of Maxwell's Equations. This set of four equations elegantly unified the principles of electricity and magnetism, predicting the existence of electromagnetic waves and fundamentally altering our understanding of light and the electromagnetic spectrum.
- Hertz's Experiment (1888): Heinrich Hertz experimentally verified the existence of electromagnetic waves predicted by Maxwell's equations. His successful generation and detection of radio waves demonstrated the practical realization of these waves and validated Maxwell's theoretical framework, paving the way for the development of wireless communication.
- Thomson's Cathode Ray Tube Experiment (1897): J.J. Thomson's experiments with cathode ray tubes provided crucial insights into the nature of electrons and their behavior in electric and magnetic fields. Thomson's work contributed to the understanding of charged particles and laid the foundation for later advancements in particle physics.
- Einstein's Photoelectric Effect (1905): Albert Einstein's explanation of the photoelectric effect, where light striking a metal surface ejects electrons, introduced the concept of photons as discrete packets of energy. This work contributed to the understanding of the particle-wave duality of electromagnetic radiation.
- Millikan's Oil Drop Experiment (1909): Robert Millikan's meticulous experiments involving charged oil droplets allowed for the determination of the elementary electric charge. His work provided experimental support for the quantization of electric charge, contributing to the precision of our understanding of electromagnetic interactions.
- Feynman's Path Integral Formulation (1948): Richard Feynman's development of the path integral formulation in quantum electrodynamics provided a powerful mathematical framework for describing the behavior of electrons and photons. This formulation has been instrumental in advancing our understanding of electromagnetic interactions at the quantum level.
- LIGO's Gravitational Wave Detection (2015): While not directly related to electromagnetic waves, the Laser Interferometer Gravitational-Wave Observatory (LIGO) experiments detected gravitational waves, which are ripples in spacetime. This groundbreaking achievement, in line with the predictions of Einstein's general relativity, represents a new frontier in the exploration of wave phenomena.
These key experiments stand as milestones in the journey of electromagnetic understanding, each contributing crucial insights that have shaped the landscape of modern physics and technology. From unraveling the nature of electric and magnetic fields to validating theoretical predictions and exploring new frontiers, these experiments exemplify the dynamic and iterative nature of scientific inquiry.
Mathematics Behind Electromagnetic Formulas: Simplifying Complex Concepts
Delving into the heart of electromagnetic theory, a profound understanding of the underlying mathematics is essential for unraveling the intricacies of electric and magnetic fields, as encapsulated by Maxwell's Equations and related formulas. This exploration unveils the mathematical foundations that simplify seemingly complex concepts, providing a clear and structured framework for comprehending the principles governing electromagnetic phenomena.
- Vector Calculus: At the core of electromagnetic theory lies the language of vector calculus. Grad, div, and curl operations become indispensable tools for expressing and manipulating electric and magnetic fields. Grasping the divergence and curl of vector fields is crucial for interpreting how electric and magnetic fields behave in different spatial configurations.
- Gauss's Law and Gauss's Law for Magnetism: Mathematics elucidates the principles encapsulated in Gauss's Law and Gauss's Law for Magnetism. Through integral calculus, these laws relate the flux of electric and magnetic fields through closed surfaces to the enclosed charges and currents, providing a quantitative link between the distribution of sources and the resulting fields.
- Faraday's Law of Induction and Ampère's Law with Maxwell's Addition: The changing magnetic field and the concept of displacement current, as incorporated in Faraday's Law and Ampère's Law with Maxwell's Addition, introduce differential calculus to describe dynamic electromagnetic processes. Understanding how these laws account for time-varying electric and magnetic fields is essential for comprehending electromagnetic wave propagation.
- Maxwell's Equations: Maxwell's Equations, a set of partial differential equations, embody the essence of electromagnetic theory. Delving into the mathematical details of these equations reveals the dynamic interplay between electric and magnetic fields, highlighting how changes in one field induce the other. The unification achieved by Maxwell's Equations serves as a pinnacle in simplifying the description of electromagnetic phenomena.
- Wave Equations and Electromagnetic Waves: The wave equations derived from Maxwell's Equations offer insights into the propagation of electromagnetic waves. These equations, often expressed in terms of partial derivatives with respect to space and time, provide a mathematical framework for understanding the speed, wavelength, and frequency of electromagnetic waves.
- Complex Numbers and Phasors: When dealing with oscillatory behavior, complex numbers and phasors become invaluable. The use of complex exponentials simplifies the representation of sinusoidal waves, enabling a concise and elegant description of electromagnetic waveforms in both time and frequency domains.
- Fourier Transforms: Fourier transforms facilitate the transition between the time and frequency domains. By decomposing complex waveforms into their constituent frequencies, electromagnetic signals can be analyzed and understood in terms of their spectral components, aiding in the design of communication systems and signal processing.
- Differential Equations in Electrodynamics: The study of electromagnetic fields often involves solving partial differential equations, reflecting the spatial and temporal variations of these fields. Differential equations serve as the mathematical bridge between theoretical formulations and real-world phenomena, allowing for the prediction and analysis of electromagnetic behavior.
- Boundary Value Problems: Solving boundary value problems, where the behavior of electromagnetic fields at interfaces between different materials is considered, introduces additional mathematical challenges. Understanding how electric and magnetic fields interact across boundaries is crucial for addressing practical scenarios in electromagnetic applications.
By mastering the mathematics behind electromagnetic formulas, students and researchers gain a powerful toolkit for deciphering the complexities of electromagnetic theory. The elegance and precision afforded by mathematical formulations not only simplify the representation of electromagnetic concepts but also serve as a gateway to innovative applications in technology, communications, and scientific exploration.
Modern Developments in Electromagnetic Research
The landscape of electromagnetic research continues to evolve, driven by technological advancements, interdisciplinary collaborations, and a deeper understanding of fundamental principles. In the contemporary scientific arena, several notable developments underscore the dynamic nature of electromagnetic research and its far-reaching implications across diverse fields. Here are some key modern developments:
- Metamaterials and Transformation Optics: Metamaterials, engineered materials with properties not found in nature, have revolutionized electromagnetic research. Researchers have developed metamaterials capable of bending light around objects, leading to the concept of transformation optics. This innovation holds promise for applications such as invisibility cloaking and improved lenses.
- Plasmonics and Nanophotonics: The study of surface plasmons, collective oscillations of electrons on metal surfaces, has given rise to the field of plasmonics. This area explores the interaction between light and nanoscale metallic structures, enabling the manipulation of light at scales much smaller than the wavelength. Nanophotonics leverages these phenomena for applications in imaging, sensing, and information processing.
- Quantum Electrodynamics (QED): Advancements in quantum electrodynamics have deepened our understanding of the interactions between light and matter at the quantum level. Precise control over individual photons and electrons is opening new possibilities for quantum information processing, quantum communication, and the development of quantum sensors.
- Terahertz Science and Technology: The terahertz frequency range, lying between microwave and infrared frequencies, has gained increasing attention for its potential applications in imaging, communication, and sensing. Researchers are exploring terahertz sources, detectors, and devices, unlocking new capabilities for non-invasive imaging and communication through materials.
- Wireless Power Transfer: Wireless power transfer technologies have advanced, allowing for the transmission of power over relatively large distances without physical connectors. Resonant inductive coupling and radio frequency (RF) energy harvesting are being explored for applications in electric vehicles, wearable devices, and the Internet of Things (IoT).
- Electromagnetic Compatibility in 5G and Beyond: As wireless communication networks transition to 5G and beyond, electromagnetic compatibility (EMC) research becomes crucial. Addressing challenges related to interference, spectrum allocation, and efficient communication in densely populated environments is essential for the success of next-generation wireless systems.
- Terahertz Imaging in Medicine: Terahertz imaging is gaining traction in medical diagnostics due to its non-ionizing nature and ability to penetrate certain materials. Researchers are exploring terahertz imaging for applications in detecting skin cancer, imaging dental structures, and studying biological tissues without the use of harmful radiation.
- Graphene and Two-Dimensional Materials: The unique properties of graphene and other two-dimensional materials have sparked interest in their applications in electromagnetics. Graphene, in particular, exhibits exceptional electrical conductivity and tunability, making it promising for applications in antennas, sensors, and transparent conductive films.
- Electromagnetic Wave Manipulation with Artificial Intelligence (AI): The integration of artificial intelligence (AI) with electromagnetic systems is a burgeoning area of research. AI algorithms are being employed to optimize the design of antennas, improve signal processing in communication systems, and enhance the performance of electromagnetic devices.
- Quantum Sensing with Electromagnetic Fields: Quantum sensors that leverage electromagnetic fields for precision measurements are a growing area of research. Quantum magnetometers and gravimeters, for instance, offer unprecedented sensitivity and are being explored for applications in navigation, geophysics, and medical diagnostics.
These modern developments underscore the interdisciplinary and dynamic nature of contemporary electromagnetic research. As technology advances and our understanding of electromagnetic phenomena deepens, the potential for transformative applications across scientific, engineering, and technological domains continues to expand.
Tips for Mastering Electromagnetic Theory as a Student
Mastering Electromagnetic Theory as a student can be both challenging and rewarding. Here are some tips and resources to help you navigate and excel in your studies:
Tips:
- Build a Strong Foundation: Ensure a solid grasp of foundational concepts in physics and mathematics, including vector calculus. A strong foundation will make it easier to understand electromagnetic principles.
- Active Learning: Engage in active learning by solving problems, working on exercises, and experimenting with concepts. Apply theoretical knowledge to real-world scenarios to reinforce your understanding.
- Visualization: Visualize electromagnetic concepts using diagrams, animations, and simulations. Visualization aids in understanding the behavior of electric and magnetic fields, making abstract ideas more tangible.
- Practice Problem-Solving: Electromagnetic theory is best learned through problem-solving. Regularly practice solving a variety of problems to reinforce your understanding and develop problem-solving skills.
- Work with Peers: Collaborate with classmates through study groups or forums. Explaining concepts to others and discussing problems can deepen your understanding and provide different perspectives.
- Use Multiple Resources: Explore different textbooks, online resources, and lecture materials. Multiple sources can provide varied explanations and examples, enhancing your comprehension.
- Relate to Real-World Applications: Relate electromagnetic concepts to real-world applications. Understanding how theory is applied in technology, communication systems, and other fields can enhance motivation and context.
- Stay Consistent: Electromagnetic theory involves intricate concepts, so consistency is key. Allocate regular, focused study sessions to avoid cramming and to reinforce long-term retention.
Conclusion
In conclusion, a solid understanding of electromagnetic theory is essential for students pursuing studies in physics, engineering, and related fields. The fundamental principles covered in this subject form the basis for comprehending a wide range of phenomena and applications, from the behavior of light to the functioning of electronic devices.
Throughout this exploration of electromagnetic theory essentials, we have delved into key concepts such as Maxwell's equations, electromagnetic waves, and the principles governing electric and magnetic fields. These concepts not only provide a theoretical foundation but also serve as the backbone for numerous technological innovations that shape our modern world.
As students, it is crucial to appreciate the interdisciplinary nature of electromagnetic theory. Its applications extend beyond theoretical physics to engineering disciplines, telecommunications, medical imaging, and much more. Mastery of these essentials opens the door to a deeper understanding of the physical world and empowers students to contribute to advancements in technology and scientific research.
In the ever-evolving landscape of science and technology, a strong grasp of electromagnetic theory not only facilitates academic success but also equips students with the tools to navigate and contribute to the dynamic fields they may choose to pursue. As you continue your academic journey, remember that the knowledge gained in electromagnetic theory serves as a valuable foundation, laying the groundwork for future discoveries and innovations.